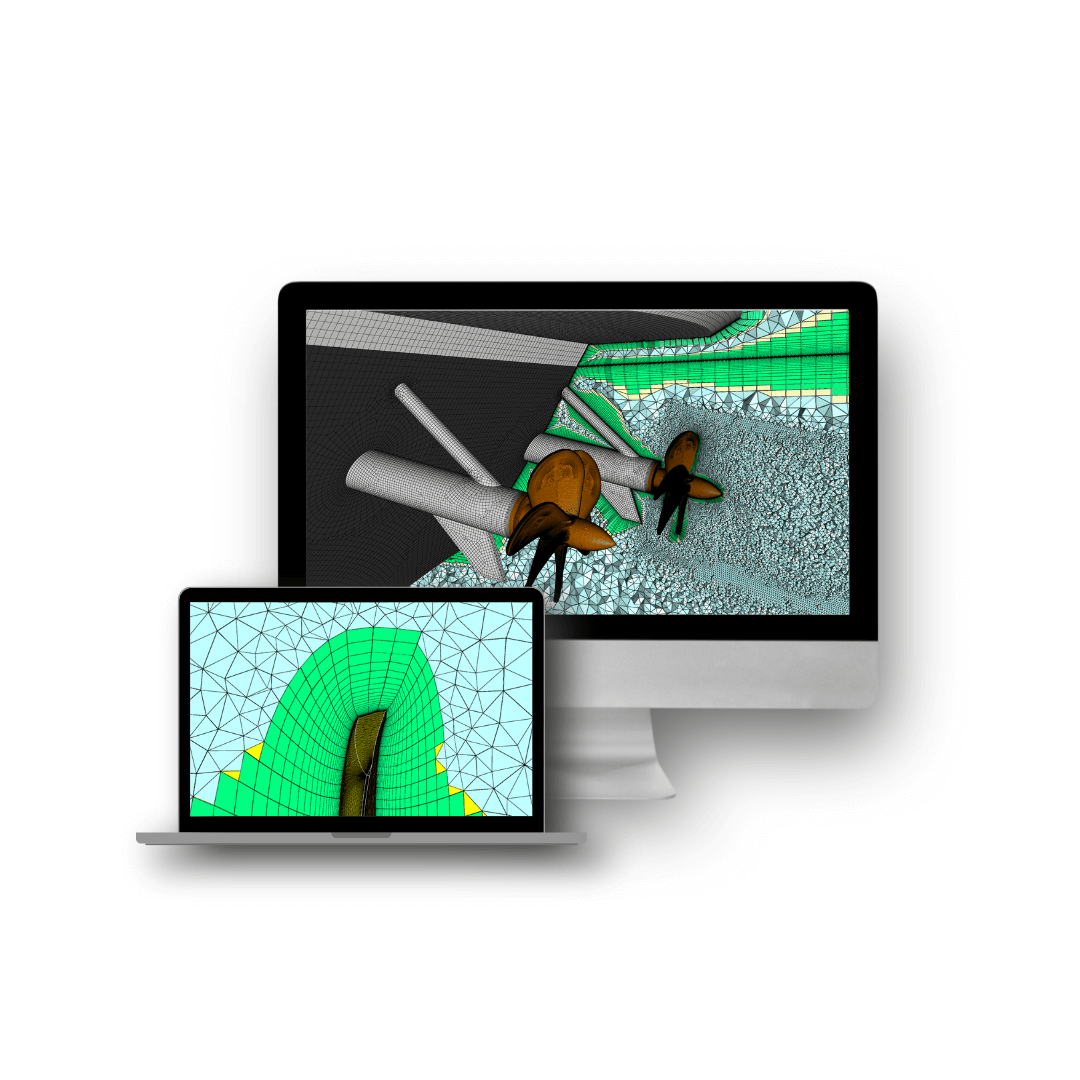
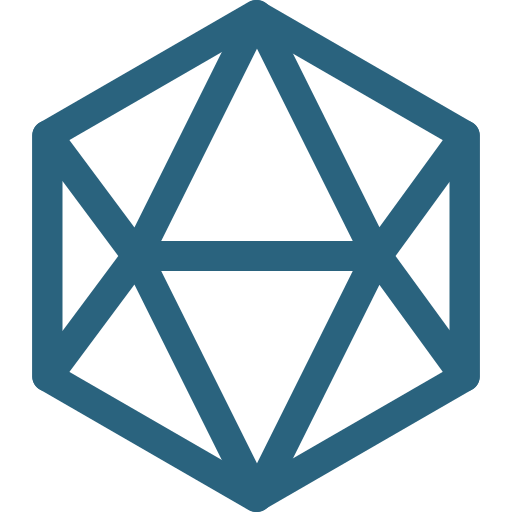
Hybrid, Overset and High-Order Meshes
Having an assortment of hexahedral, overset, and high-order cell definitions facilitates rigorous sensitivity and quality analysis when mesh sizes can reach hundreds of millions or even billions of cells.
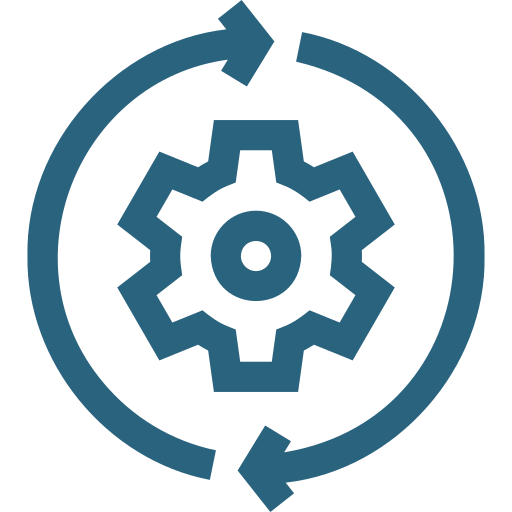
Ease of Interface With Any Simulation Workflow
Fidelity Pointwise empowers CFD users to access top-quality meshes, while excelling in the seamless import and export of geometry models and software tools, ensuring an efficient workflow.
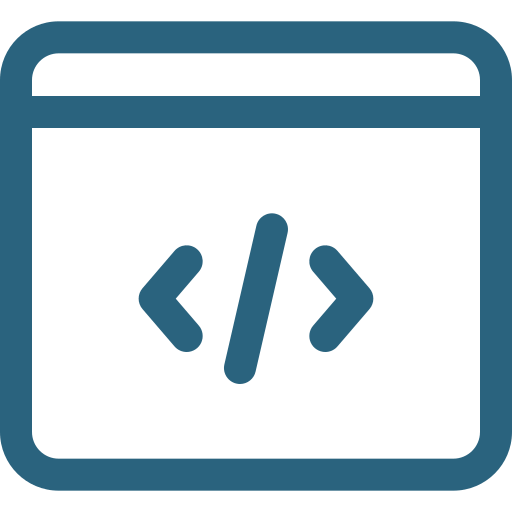
Customizable Scripting and Templates
You can streamline your repetitive meshing tasks scripting your taylor-made routine within Fidelity Pointwise environment.